Introduction
This study was concerned with the design, assembly, and operation of a pyroelectric converter for harvesting waste heat by performing the Olsen cycle on 60/40 P(VDF-TrFE) thin films. First, the underlying physical principles were presented. Then, the pyroelectric device assembled and the associated thermal and electrical sub-systems were described in details. Finally, the experimental results were reported and discussed. The following conclusions can be drawn:
Thermal Sub-System
- 60/40 P(VDF-TrFE) pyroelectric thin films (30-70 microns thick).
- Electrodes: Al films (1 micron thick).
- P(VDF-TrFE) films were placed between mica plates.
- Enclosure cylinders: Teflon (inner and outer diameters of 38.10 and 57.15 mm).
- Working fluid: 50 cst silicone oil.
- Heat source: 0.2 mm thick 50 W Kapton electrical heater taped to 0.79 mm thick copper heat spreader
- Cold heat exchanger: tap water fed by a DC pump and flowing through a 3.18 mm OD copper coil with overall length of 34.3 cm.
- Thermal insulation: glass wool.
- Silicone gaskets were placed between enclosing cylinders to prevent leaks.
- Each pyroelectric element in the pyroelectric assembly was sandwiched between two mica plates (310 microns thick)
- 10 mm x 10 mm window was cut away in the mica plates' center.
- Four pieces of 89 microns thick Kapton tape were used to tape the edges of the pyroelectric element to the mica plate.
- The stack consisted of 38 pyroelectric elements separated by 330 microns wide microchannels.
|
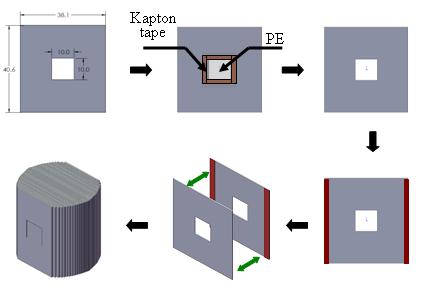
Pyroelectric stack assembly
|
|
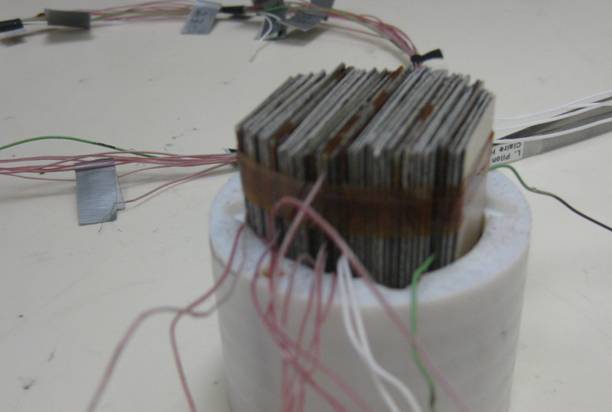
Stack inside the device
|
Electrical Sub-System
Poling and Energy Harvesting Circuit Performing the Olsen Cycle
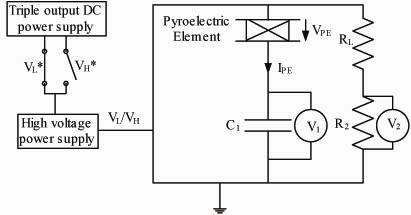
-
a pyroelectric element made of a 60/40 P(VDF-TrFE) thin film with its electrodes
-
a triple output DC power supply model 1651 from BK Precision
-
a high voltage power supply model PS325 from Stanford Research Systems Inc.
-
V SRS ~ 860 V, 0.1 < V <10 V, C 1 = 1.0 
-
two 10A 125 VAC rated rocker switches model RRA22H3BBBNN by Cherry Electrical Products (WI, USA).
-
resistors R 2 = 21.8  and R L = 7.80
-
an metallized polypropylene film capacitor model ECWF2105JB by Panasonic Corp., Japan
Instrumentation
Temperature and Pressure Measurements
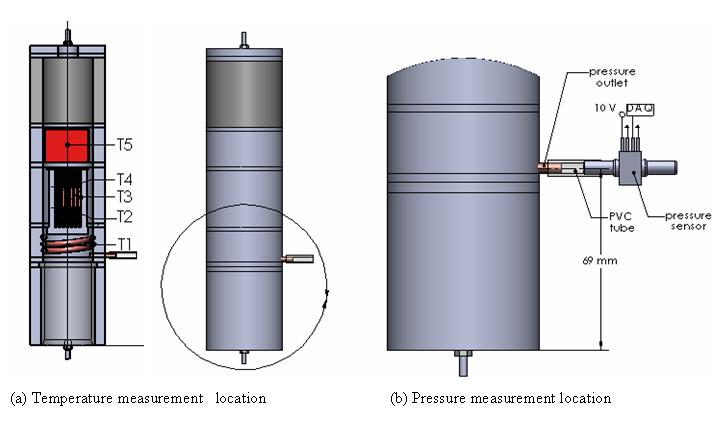
Temperature and Pressure as a Function of Time
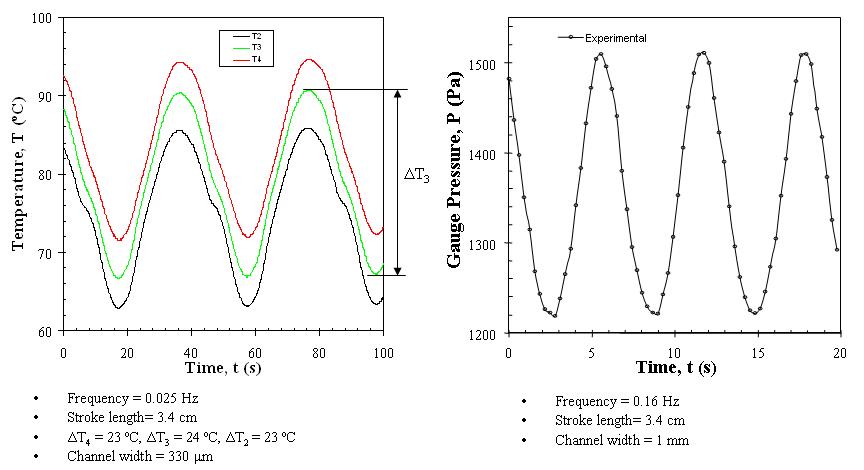
|
|
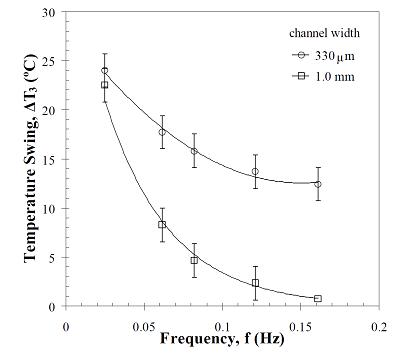
Temperature swing in the middle of the pyroelectric element as a function of frequency and channel width. |
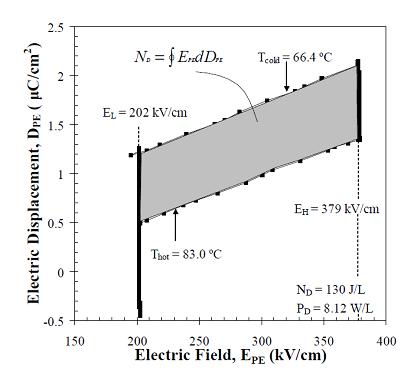
Olsen cycle performed experimentally with EL = 202 kV/cm and EH = 379 kV/cm. |
Energy and Power Densities versus High Electric Field
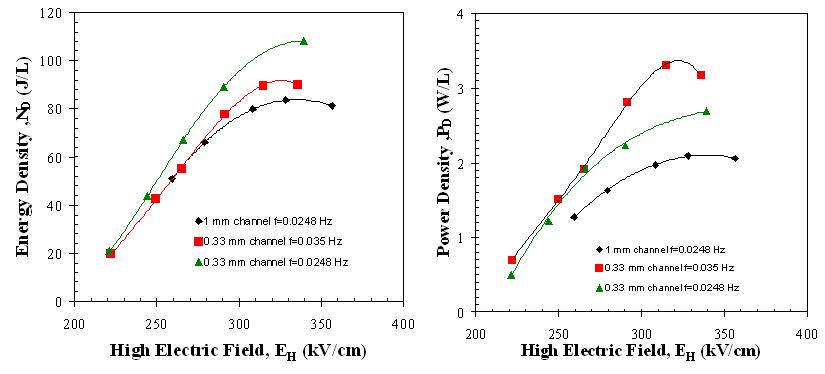
Effect of the high electric field EH on the device performances with EL = 202 kV/cm.
|
Conclusions
-
Temperature swing at the pyroelectric element decreased as frequency increased for constant arithmetic mean temperature at the center of the pyroelectric element around 74°C.
-
For the same frequency, stroke length, and the mean temperature, temperature swing at the pyroelectric element increased as the channel width decreased.
-
Heat provided by the electrical heater to maintain the mean temperature at the center of the pyroelectric element at 74°C increased as stroke length and frequency increased.
-
In order to maximize the pyroelectric element energy and power densities, it was best to operate the device where the arithmetic mean temperature at center of the pyroelectric element was equal to 74°C.
-
Operating the device at an arithmetic mean temperatures larger than 74±1°C caused a larger current leakage across the PE while operating the device at an arithmetic temperatures smaller than 74°C prevented the PE from undergoing the ferro- to paraelectric phase transition.
-
The optimum low and high electric fields EL and EH in the Olsen cycle were 202 kV/cm and 379 kV/cm, respectively.
-
The largest energy density achieved by the device was 130 J/L of pyroelectric material at 0.061 Hz. To the best of our knowledge, this is the largest energy density achieved by any pyroelectric energy converter using polymeric pyroelectric material P(VDF-TrFE).
-
The largest power density was 10.7 W/L of pyroelectric material at 0.12 Hz.
Future research should focus on further reducing the channel width to increase the temperature swing and the number of pyroelectric elements in the stack to increase the power density and the efficiency. Synthesize or search for other pyroelectric materials that have: (i) large electric break down field Eb, (ii) electrical resistivity larger than 0.3 at 85°C, (iii) Curie temperature between 30 and 80°C, and (iv) energy density ND > 282 J/L when dipped between hot and cold baths. Moreover, the material must be cost effective compared to that of 60/40 P(VDF-TrFE) which costs approximately $5000/kg or $0.90/kW using the present device.
Publications
H.T. Nguyen, A. Navid, and L. Pilon, 2010. Pyroelectric Energy Converter for Waste Heat Energy Harvesting Using Co-Polymer P(VDF-TrFE) and Olsen Cycle. Applied Thermal Engineering, Vol. 30, pp. 2127-2137. 10.1016/j.applthermaleng.2010.05.022
|